Forschung
Molecular mechanisms of dendrite patterning
Neurons come in different shapes and flavors, yet each sub-type typically features highly stereotyped dendrite morphology. Dendrites are further often arranged in layers and display self-avoidance (no overlap of dendrites belonging to the same neuron). In addition, subsets of neurons including retinal ganglion cells in mammals as well as nociceptive neurons in Drosophila larvae feature space-filling receptive fields with minimum overlap, a phenomenon termed tiling. How these patterning mechanisms are controlled by molecular cues is therefore a subject of intensive studies.
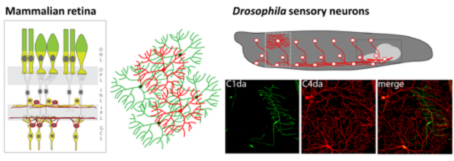
Work on larval sensory neuron development has significantly contributed to the identification of key molecules and mechanisms regulating basic dendrite patterning. These include Dscam, a cell surface receptor required for self-avoidance, as well as integrins and Semaphorin/Plexin, which mediate ECM adhesion and layer formation to ensure self-avoidance.
In our recent work we have identified the neuronal receptor tyrosine kinase Ret, which is required for the formation of space-filling dendritic fields of Drosophila nociceptive neurons (Soba et al., Elife 2015; Hoyer et al., Cell Rep. 2019). We are studying its upstream and downstream signals, which promote and stabilize dendrite growth and adhesion. Ret function enables spreading of dendrites to establish complete receptive field coverage. These studies allow us to gain mechanistic insight into the basis of dendritic field development and patterning, which is essential for neuronal network function.
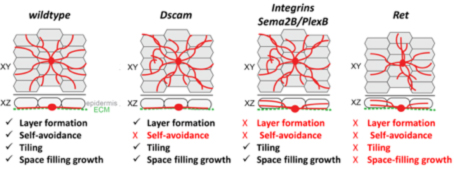
Maintenance of circuit integrity and synaptic specificity
Juvenile organisms like Drosophila larvae maintain the functional connectivity of their network through all stages, while increasing their body surface 100-fold within few days. This requires immense growth of dendrites and circuit-specific synapse addition to maintain receptive field coverage and functional output, respectively.
How is this coordinated growth regulated at the organismal and cellular level? We have identified two distinct classes of regulators, which are required to sustain neuronal morphology and synaptic connectivity of sensory neurons.
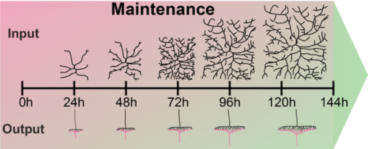
The first class is required for coordinated growth to keep neuronal and synaptic growth rates in sync with larval development. Genes regulating this process also seem to be required for circuit specific addition of synapses (Tenedini et al., Nat. Comm 2019, Hu et al., J Neurosci. 2020). We are investigating the underlying signaling machinery to gain mechanistic insight into the regulation of growth synchronization and synaptic specificity. This work is of particular relevance as abnormal connectivity has been linked to neurodevelopmental disorders including autism and schizophrenia.
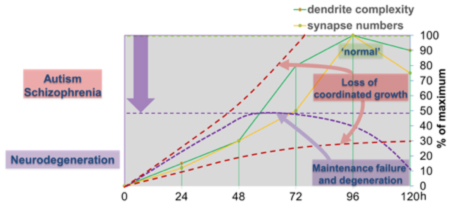
We have also identified a second class of regulators, which are required to sustain neuronal morphology and synaptic connectivity of sensory neurons during larval development. The loss of these genes results in maintenance failure and degeneration suggesting a general failure of basic cellular functions underlying maintenance of neuronal morphology and connectivity. We investigate potentially common and diverging pathways to gain conceptual understanding of circuit maintenance, degeneration, and its reversal.
Neuromodulatory control of behavior
Circuits need to be functional to sustain behavior, and most larval behaviors are consistently maintained during development. This is particularly true for nociception, which is an evolutionary conserved mechanism to detect and avoid noxious stimuli. Similarly to vertebrates, Drosophila larval nociceptors are able to detect different modalities including nociceptive touch and heat. How behavioral responses to these distinct modalities are encoded at the network level has so far not been elucidated. We have recently uncovered a circuit and neuromodulatory mechanism encoding mechano-nociceptive escape behavior (Hu et al., Nat. Neurosci. 2017), which relies on the Drosophila Neuropeptide Y homolog sNPF. sNPF action on the identified circuit elements is specific to mechano-nociception, while thermo-nociception seems to be encoded by a different part of the network. We recently showed that larval avoidance of noxious light (UV and blue light) depends on a similar circuit that relies on peptidergic feed-forward signaling (Imambocus et al., Curr. Biol. 2022). Thus, sensory processing in the larval nociceptive network utilizes peptidergic action on top of its neuronal network to select appropriate escape behavior.
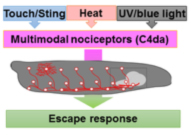
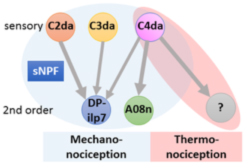
We are continuously investigating how neuromodulation regulates circuit function by developing novel tools and using molecular, optogenetic and behavioral approches. Overall, our studies aim to shed light on neuropeptide action in network function and behavioral action selection.